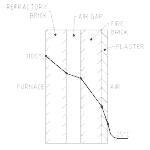
Heat is energy and its nature is to flow from a state of high excitement to one of low excitement. Heat is transferred from a hot place to a cold place by convection, conduction or radiation. This article explains the three modes of heat transfer and provides simple examples of each. Methods to reduce and increase heat transfer are also presented.
Keywords: emissivity, black body, absorptivity, reflectivity, transmissivity
Overview of the Heat Transfer Modes
Radiation is the transfer of heat energy by the movement of electro-magnetic radiation through open space. All matter in the universe radiates energy unless its temperature is at absolute zero (-273 °C). Emitted radiation will be absorbed into, reflected from or transmitted through a body in the path of the radiation.
Conduction is the transfer of heat from one part of a substance to another, or to second substance in contact with it. Heat energy moves through a body by exciting neighbouring atoms. A temperature gradient (slope) is created from the hotter to the cooler sections.
Convection is the transfer of heat within a fluid (liquid or gas) by the mixing of one portion of fluid with another. The mixing can be by changes in density (e.g. hot air rising over an open fire) and is called natural convection or by mechanical means (e.g. a room fan heater) and is then known as forced convection.
Radiation in more detail
When radiated energy from a hot body hits another body the portion of energy that is absorbed by the second body is called its absorptivity. The amount absorbed is not usually the total amount of energy that hits it. Of the energy that is not absorbed, some of it is reflected away by the body. The portion reflected away is called the body’s reflectivity. The second body can also transmit energy through itself and the portion that is transmitted straight through is called its transmissivity.
Should it be possible for a surface to totally absorb all the energy that hits it without reflection or transmission it would be called a ‘black body’. A ‘black body’ is a surface that takes in all energy and emits nothing. Snow is nearly a ‘black body’ to heat radiation. Snow absorbs 98.5% of the heat that falls on it. Outer space is a ‘black body’ to the sun and absorbs all the sun’s energy.
The ratio of the energy absorbed by a ‘black body’ to the energy absorbed by a ‘real’ body is called the ‘real’ body’s emissivity. The more ‘black body’ like a ‘real’ body behaves, the higher its emissivity. A ‘black body’ has an emissivity of 1. In the case of snow its emissivity to heat is nearly 1 as it absorbs most heat. The higher the emissivity of a surface the greater the amount of energy it absorbs and the less the amount of energy reflected or transmitted through.
Surface finish affects how much the surface behaves like a ‘black body’ (the emissivity). Rough surfaces are better absorbers and emitters of heat than smooth surfaces. A polished surface is a good reflector and a poor absorber so its emissivity is low.
The temperature of a body greatly affects the energy it emits. A body at a higher temperature releases more energy than the same body at a lower temperature. The value of the energy emitted by a surface is given by the Stefan-Boltzmann Law e quation below.
$$ \displaystyle\large E=5.67\times {{10}^{-8}}\varepsilon {{T}^{4}}\text{ }{\text{Watt}}/{{{\text{m}}^{\text{2}}}}\;$$
Where ‘ε’ is the body’s emissivity and ‘T’ is the temperature in Kelvin (Kelvin = Centigrade + 273). The higher its temperature the more the energy is emitted.
The equation also tells us that surfaces with low emissivity emit less energy and so make good energy reflectors. Aluminium has low emissivity and it is why polished aluminium foil is often used to shield surfaces that are at temperatures different to their surroundings.
Conduction in more detail
The amount of total heat transferred through a substance (conduction) depends on:
- the surface area ‘A’ exposed to the heat (more areameans more energy is available),
- the willingness of the substance to transmit the heat.
This is called its thermal conductivity ‘k’. Each material has a different ability to resist heat transfer through itself. A material with a high resistance to heat transfer has a low thermal conductivity. One with little resistance has a high thermal conductivity and willing lets heat move through itself. Copper with thermal conductivity of 386 W/m °C is 9000 times more willing to transmit heat than cork with a thermal conductivity of 0.043 W/m °C. - the thickness of the body ‘x’ (the thicker the material the less heat gets through it to the other side),
- the temperature difference between one side of the body at ‘t1’ and the other side at ‘t2’ (greater temperature differences cause more energy transfer).
The equation for conduction of heat through a flat surface is shown below:
$$ \displaystyle\large Q=\frac{kA\left( {{t}_{1}}-{{t}_{2}} \right)}{x}\text{ Watt}$$
The equation shows that as either thermal conductivity, or the surface area, or the temperature difference between two positions increases, then the heat transferred also rises. However as the distance between two points increases the heat transfer between the two positions drops.
Convection in more detail
Heat Transfer from a Solid to a Fluid
A hot surface will transfer heat to a fluid (liquid or gas). In order to boil water for a cup of tea or coffee, the energy from a kettle’s heating element must be transferred to the water. The water nearest the element touches the surface and gets hot by contact (conduction). Natural convection causes the hot water to rise and new, cooler water touches the element and gets heated.
The heat transfer rate depends on a property of the fluid called its heat transfer coefficient ‘h’ in W/m2 oC. The higher the heat transfer coefficient the easier it is for heat to flow through the fluid.
The heat transfer from a solid of surface area ‘A’ at a particular temperature, to a fluid at a different temperature touching it, is given by the equation below:
$$ \displaystyle\large Q=hA\left( {{T}_{surface}}-{{T}_{fluid}} \right)\text{ Watt}$$
The equation tells us that convection heat transfer can be increased by having more surface area in contact with the fluid, or by increasing the heat transfer coefficient or by having a higher temperature difference between the surface and the fluid.
Convection can also be by mechanical means (known as forced convection) such as pumping through heat exchangers, using static mixers inside tubes to make the flow turbulent and putting agitators in steam jacketed tanks. Forced convection is used because the fluid is forced into contact with the hot surface many times during its passage around the heat transfer equipment. This increases the heat input and warms the fluid faster.
Heat Loss Minimisation
Where heat loss is to be reduced it becomes necessary to:
- reduce heat transfer coefficients,
- reduce thermal conductivity (or increase thermal
resistance), - use low emissive surfaces,
- increase the thickness of the walls,
- keep surface areas small,
- block the direct radiation, conduction or convective
paths between the two substances, - minimise the temperatures of surfaces,
- minimise temperature difference with the surroundings.
Radiated heat whose escape is to be minimised (like the flame in a boiler) is usually ‘boxed-in’ with thick walls made of low conducting materials like refractory bricks. These are then insulated on the outer side with low conducting, low emissive materials like fiberglass blankets. This sandwich construction offers high resistance to heat transfers (i.e. low conductivity). For example, the flame temperatures in a boiler maybe 1000 °C (588 °F) but the outside wall can be touched by hand.
An example of the use of multiple materials to create a thermal resistance is shown in Figure No. 1. It is a cross- section through a furnace wall showing the temperature of the various surfaces that make-up the wall (the temperature gradient). Note that the air gap stops conduction as the main heat transfer mode and makes radiation the dominant mode. If fiberglass batts could be used to fill the air gap, or aluminium metal sheeting hung between the walls, radiation would have greatly reduced and the outside wall temperature would be even lower.
In a steam jacketed tank the inside wall is made as thin as possible to reduce the thermal resistance. The outside wall of the jacket is insulated to prevent heat loss to the cold surroundings. If it were not insulated the outer wall of the steam jacket would radiate heat into the cold surroundings.
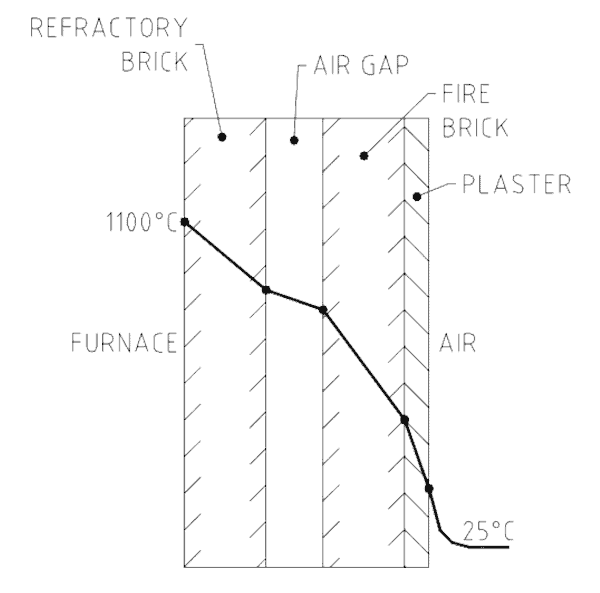
Heat Loss Maximisation
In situations where maximum transfer of heat is required it becomes necessary to find ways to:
make the fluid contact the hot surfaces as much as possible by using turbulence,
- keep the temperature difference between the surface and the fluid as great as possible,
- keep the thermal resistance of materials separating cold fluids from hot fluids the as low as possible,
- get the heat transfer coefficient of the fluids up,
- remove any surface scaling,
- use materials of high thermal conductivity (i.e. low thermal resistance).
For example, boiling a pot of water over an open fire in the forest takes longer than boiling the same pot over a fireplace hearth in a house and both take longer that boiling the same amount of water in an electric kettle.
Out in the open air, the pot gets only a small amount of the fire’s heat. Most is radiated in all directions. Some radiation hits the pot and some convective heat from the hot rising air touch the pot. In the hearth the pot gets radiation off the hot surrounding bricks and the fire and also convection heat from the hot rising air. In the kettle the water gets heat from direct contact with the heating elements. All the heat in the kettle’s heating elements is injected into the water and little is lost to the surroundings.
When trying to heat-up a substance it is necessary to insure that the maximum heat possible is put into it and not lost to the surroundings.
Mike Sondalini – Equipment Longevity Engineer
Reference: Applied Thermodynamics for Engineering Technologists, T. D. Eastop. A. McConkey, Longman
If you found this interesting, you may like the ebook Process Control Essentials.
Leave a Reply